Therapeutic Effects of Umbilical Cord Blood Derived Mesenchymal Stem Cell-Conditioned Medium on Pulmonary Arterial Hypertension in Rats
Article information
Abstract
Background:
Human umbilical cord blood-derived mesenchymal stem cells (hUCB-MSCs) may have multiple therapeutic applications for cell based therapy including the treatment of pulmonary artery hypertension (PAH). As low survival rates and potential tumorigenicity of implanted cells could undermine the mesenchymal stem cell (MSC) cell-based therapy, we chose to investigate the use of conditioned medium (CM) from a culture of MSC cells as a feasible alternative.
Methods:
CM was prepared by culturing hUCB-MSCs in three-dimensional spheroids. In a rat model of PAH induced by monocrotaline, we infused CM or the control unconditioned culture media via the tail-vein of 6-week-old Sprague-Dawley rats.
Results:
Compared with the control unconditioned media, CM infusion reduced the ventricular pressure, the right ventricle/(left ventricle+interventricular septum) ratio, and maintained respiratory function in the treated animals. Also, the number of interleukin 1α (IL-1α), chemokine (C-C motif) ligand 5 (CCL5), and tissue inhibitor of metalloproteinase 1 (TIMP-1)–positive cells increased in lung samples and the number of terminal deoxynucleotidyl transferase–mediated deoxyuridine triphosphate nick-end labeling technique (TUNEL)–positive cells decreased significantly in the CM treated animals.
Conclusions:
From our in vivo data in the rat model, the observed decreases in the TUNEL staining suggest a potential therapeutic benefit of the CM in ameliorating PAH-mediated lung tissue damage. Increased IL-1α, CCL5, and TIMP-1 levels may play important roles in this regard.
Pulmonary artery hypertension (PAH) is a progressive chronic disease with a high mortality rate [1]. PAH has a complex disease mechanism, but its cardinal signs are an elevation of pulmonary artery pressure, right ventricular (RV) hypertrophy, and arteriolar wall remodeling [2]. Increased pulmonary vascular resistance and over-proliferation of pulmonary artery endothelial cells leads to remodeling of the pulmonary vasculature [3-5]. There is also damage to the pulmonary microvasculature impacting the blood flow from the heart to the lungs [6,7]. Although current treatments may prolong and improve quality of life for the patients, the long-term prognosis for PAH is poor with a 2- to 3-year survival at the time of diagnosis [1].
Autologous implantation of bone marrow mononuclear cells, known to be enriched in mesenchymal stem cells (MSCs), has demonstrated safety and effectiveness in therapeutic angiogenesis [8]. A number of studies have also indicated a therapeutic benefit from bone marrow derived MSCs in increasing respiratory function in animal models of PAH [9,10]. In separate studies, human umbilical cord blood-derived MSCs (hUCB-MSCs) have also improved lung function in animal models of PAH and in a number of human PAH patients [11-13].
In previous studies, we demonstrated the neuroprotective potential of various conditioned media (CM), namely human adipose tissue-derived stem cell (hADSC)–conditioned media and human neural stem cell (hNSC)–conditioned media to treat rats with stroke and Huntington’s disease [14,15]. We also investigated gene expression changes by microarray analysis after injection of hUCB-MSCs into rats in an experimental model of PAH [16]. Based on our findings from that study, we undertook an investigation to assess the feasibility and safety of conditioned medium from hUCB-MSCs (hUCB-MSC-CM) in the same rat PAH model. We also tested the hypothesis that the conditioned media from these cells may lead to improved lung function in the affected rats. Here, we elaborate on our results and demonstrate that the conditioned media provides a therapeutic benefit in the rat model of PAH. As there are certain advantages in using conditioned media in lieu of autologous whole bone marrow or umbilical cord cells as sources for MSCs, our data may be provide a means of increasing the accessibility of MSCs to treat various diseases including PAH.
MATERIALS AND METHODS
Animals
Six-week-old male Sprague-Dawley rats were used. All rats were housed in climate-controlled conditions with a 12-hour light/12-hour dark cycle, and had free access to food and water. All animal experiments were approved by the appropriate Institutional Review Boards of the Seoul National University College of Medicine (Seoul, Korea; SNU-101122-2) and conducted in accordance with National Institutes of Health Guide for the Care Use of Laboratory Animals (NIH publication No. 86-23, revised in 1996).
Pulmonary arterial hypertension rat model
PAH was induced by subcutaneous injection of 50 mg/kg monocrotaline (MCT; Sigma-Aldrich, St. Louis, MO, USA) dissolved in 0.5 N HCl. The rats were grouped into a control group (C group) (n=20), injection of α–minimal essential medium (αMEM) followed by MCT group (M group) (n=20), and injection of MCT followed by hUCB-MSC-CM transfusion group (CM group) (n=20). αMEM and hUCB-MSC-CM (0.5 μL/hr) were transfused by tail-vein 7 days after MCT injection. The animals were sacrificed at 7, 14, 21, and 28 days after hUCB-MSC-CM transfusion. Tissues were removed and immediately frozen at –70°C for enzyme analysis.
Cell preparation and culture of hUCB-MSCs
hUCB-MSCs were obtained from the Biomedical Research Institute (Seoul, Korea). Isolated human MSCs were expanded in culture as previously described [6]. hUCB-MSCs were maintained in αMEM (Gibco, Grand Island, NY, USA) supplemented with 10% fetal bovine serum (Gibco), 100 U/mL penicillin (Gibco), and 100 g/mL streptomycin (Gibco). Passages up to 5 were used for experiments.
Preparation of hUCB-MSC-CM
To generate hUCB-MSC-CM spheroids [16,17], 30 μL of cell suspension (1×106 cells/mL) were applied to the lid of a Petri dish containing phosphate buffered saline (PBS). After 24 hours of incubation, spheroids formed in the drops were retrieved. For the three-dimensional bioreactor culture, hUCB-MSC spheroids (4.2×107 cells) were cultured in a siliconized spinner flask (Bellco, Vineland, NJ, USA) containing 70 mL αMEM with stirring at 70 rpm. To obtain CM, the medium was changed to αMEM without serum, and the cells were cultured for 2 days. CM was collected by centrifugation [14].
Determination of the organ weights and right hypertrophy index
The rats were weighed and observed for general appearance during the study period. The animals were sacrificed at the scheduled time. The wet weights of the excised right ventricle (RV), left ventricle (LV), and interventricular septum (IVS) were measured. The weights of the LV and IVS were added (LV+IVS) to determine the RV to LV+IVS ratio [RV/(LV+IVS)], which was used to determine the right hypertrophy index.
Pulmonary hemodynamics
Rats were anaesthetized by intraperitoneal injection of urethane and secured on a surgical stage. An 8-mm-long right internal jugular vein was isolated and ligated at the distal end. The vessel was cut at the proximal end of ligation. A catheter filled with heparinized saline was rapidly inserted along the incision and slowly advanced for about 5 cm to enter the pulmonary artery. The standard of pulmonary hypertension was defined as a systolic pulmonary pressure (SPAP) larger than 50 mm Hg [18]. Hemodynamic parameters were recorded at baseline and at 7, 14, 21, and 28 days.
Immunohistochemistry
Excised lung tissues were incubated overnight in 10% buffered formalin. Four-micrometer-thick sections were cut from paraffin embedded tissue blocks, deparaffinized in xylene, and rehydrated in graded alcohol solutions (70%–100%). Heat antigen retrieval was achieved by boiling the tissue sections in antigen retrieval solution in pH 6.0 or pH 9.0 (Dako, Carpinteria, CA, USA) for 10 minutes in a microwave prior to incubation at 4°C overnight with primary antibodies against interleukin 1α (IL-1α), chemokine (C-C motif) ligand 5 (CCL5), and tissue inhibitor of metalloproteinase 1 (TIMP-1; Abcam, Cambridge, MA, USA). After incubation with the appropriate biotinylated secondary antibodies for 30 minutes at 4°C and subsequently with streptavidin (Dako, Kyoto, Japan), color development was done using diaminobenzidine (DAB) as a chromogen and counterstained with hematoxylin.
Western blot analysis
The tissue was homogenized in 10 mM Tris HCl buffer, pH 7.4 containing 0.5 mM ethylenediaminetetraacetic acid, pH 8.0, 0.25 M sucrose, 1 mM phenylmethylsulfonyl fluoride, 1 mM Na4VO3, and a protease inhibitor cocktail (Roche-Boehringer-Mannheim, Mannheim, Germany). After centrifugation, the supernatant was subjected to sodium dodecyl sulfate polyacrylamide gel electrophoresis (SDS-PAGE). Samples equivalent to 25 μg of protein content were loaded and size-separated by 8%–12% SDS-PAGE. The proteins on the acrylamide gel were transferred to a polyvinylidene difluoride membrane (Millipore, Bedford, MA, USA) at 400 mA in a transfer buffer containing 25 mM Tris and 192 mM glycine, pH 8.4. The nitrocellulose membrane was blocked in tris-buffered saline with 5% non-fat dry milk at room temperature for 1 hour in 0.1% Tween 20 and incubated with the appropriated primary antibodies, including anti–IL-1α (Santa Cruz Biotechnology, Santa Cruz, CA, USA), anti-CCL5 (Fitzgerald Industries International, Concord, MA, USA), anti–TIMP-1 (Abcam), and anti-caspase-3, anti-Bcl-2, anti-actin (Santa Cruz Biotechnology) at 4°C for overnight. The membranes were then incubated with horseradish peroxidase-conjugated secondary antibody (Cell Signaling Technology, Danvers, MA, USA) for 1 hour at room temperature. After washing, the membranes were visualized by a chemiluminescent ECL-detection kit from GE-Healthcare (Piscataway, NJ, USA).
Cytokine array and gene expression in lung tissues
The lung samples were collected at termination (4 days after hUCB-MSC-CM injection) and quickly frozen in liquid nitrogen. A rat cytokine array (ARY008, R&D Systems, Minneapolis, MN, USA) was used to screen the lung homogenates according to the manufacturer’s instructions. The samples were pooled per treatment group and equal amounts of protein were loaded on the blots.
In situ terminal deoxynucleotidyl transferase–mediated deoxyuridine triphosphate nick-end labeling technique assay for lung cell apoptosis
Apoptotic cells in the tissue sections were detected by the terminal deoxynucleotidyl transferase-mediated deoxyuridine triphosphate nick-end labeling technique (TUNEL) using a commercial apoptosis kit (TACS TM TdT Kit, R&D Systems), according to the supplier’s instructions. In brief, the lung tissue sections were de-paraffinized with xylene and ethanol and rinsed with PBS. The sections were then treated with proteinase K in PBS followed by quenching of endogenous peroxidase. A biotinylated dNTP mix was added to the 3’-OH ends of DNA by terminal deoxynucleotidyl transferase (TdT). After incubating with streptavidin-horseradish peroxidase, the sections were stained with DAB and counterstained with methyl green. Finally, the sections were dehydrated in ethanol, cleared with xylene, and mounted with coverslips in a permanent medium. According to the supplier’s instructions, experimental controls included for the assay were TACS-nuclease–treated thyroid tissue sections as the positive control and the omission of the TdT reaction step as the negative control.
Statistical analyses
Results were expressed as the mean±standard deviation. An unpaired two-tailed t test and Mann-Whitney test were used, and a p-value less than .05 was considered statistically significant. SPSS ver. 14.0 for Windows (SPSS Inc., Chicago, IL, USA) was used for all statistical analyses.
RESULTS
Changes in body and organ weights and systolic pulmonary artery pressure after injection with hUCB-MSC-CM in PAH rats
hUCB-MSC-CM has the potential to increase cell differentiation and induce immune modulation in various disease models [19,20]. However, the role of hUCB-MSC-CM in PAH has not been well elucidated. To address this, in our rat model of PAH, following MCT treatment, we treated rats with hUCB-MSC-CM and sham treated for the control group. There was a significant decrease in body weight at 14, 21, and 28 days in the MCT group (M group) compared to the control group (C group). However, body weight increased at 21 and 28 days in the conditioned media treated group (CM group) compared to the M group. The M group also showed increased weights of the RV at 21 and 28 days. The sum weight of LV+IVS was not significantly different between the C, M, and CM groups at the time point tested. The ratio of RV to LV+IVS, namely RV/LV+IVS, was significantly higher at 14, 21, and 28 days in the M group compared with the C group. However, the RV/LV+IVS ratio was significantly decreased at 28 days in the CM group compared with the M group. Also, LV+IVS was significantly lower in both M and CM groups compared to the C group at 14, 21, and 28 days. The lung weight was significantly increased in the M group compared with the C group at 21 and 28 days. However, the lung weight was significantly decreased in the CM group compared to the M group at 28 days (Table 1). The mean SPAP was also significantly increased in the M group compared to the C and CM groups at 14, 21, and 28 days (Table 2).
Cytokine profile in the lung tissues after hUCB-MSC-CM treatment
A profile of the cytokines in the lung homogenates was made to investigate potential changes from hUCB-MSC-CM treatment (Fig. 1). Ten pro-inflammatory cytokines that included cytokine-induced neutrophil chemoattractant-1 (CINC-1), cytokine-induced neutrophil chemoattractant-2a/b (CINC-2a/b), chemokine (C-X-C motif) ligand 1 (CX3CL1), lipopolysaccharide-induced CXC chemokine (LIX), leukocyte endothelial cell adhesion molecule 1 (LECAM-1), chemokine (C-X-C motif) ligand 7, TIMP-1, vascular endothelial growth factor (VEGF), IL-1α, and CCL5 were examined in the C, M, and CM groups. CINC-1, CINC-2a/b, CX3CL1, LIX, LECAM-1, TIMP-1, and VEGF were lower in the M and CM groups, whereas TIMP-1, IL-1α, and CCL5 were higher in the CM group compared to the C and M groups. CCL7 was higher in the M group, whereas CCL7 was lower in the CM group compared to the M group (Fig. 1).
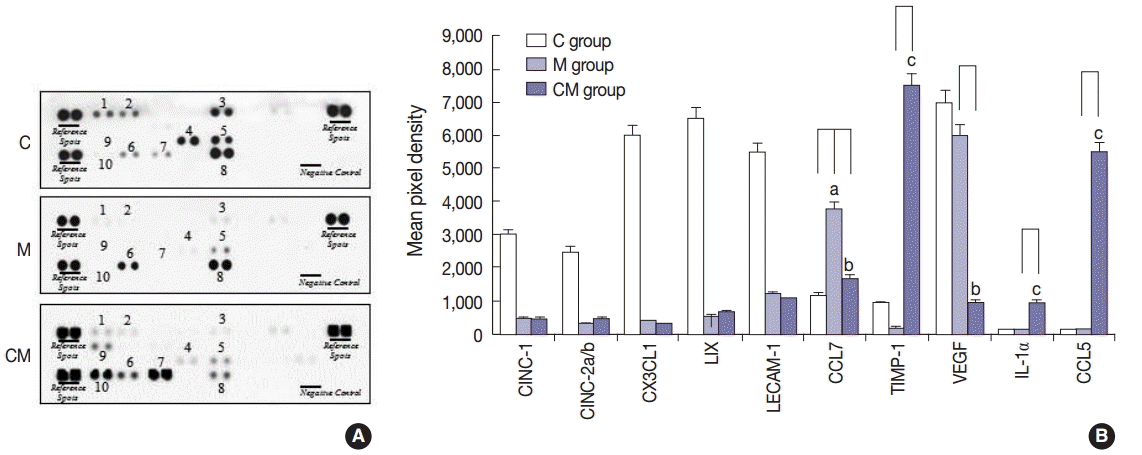
Inflammatory cytokine expressions in the three groups. (A) To screen whether hUCB-MSCs-CM affect local production of inflammatory cytokines by lung cells in the three groups, a cytokine array is performed on lung homogenates. (B) TIMP-1, IL-1α, and CCL5 are higher in the CM group compared to the C and M groups, whereas CCL7 and VEGF are lower in the CM group compared to the M group. CINC-1, CINC-2a/b, CX3CL1 LIX, and LECAM-1 are higher in the C group compared to the M and CM groups. C group, control group (n = 7); M group, monocrotaline group (n=7); CM group, hUCB-MSCs-CM group (n=7). hUCB-MSCs-CM, conditioned medium from human umbilical-cord blood derived mesenchymal cells; CINC-1, cytokine-induced neutrophil chemoattractant-1; CINC-2a/b, cytokine-induced neutrophil chemoattractant-2a/b; CX3CL1, chemokine (C-X-C motif) ligand 1; LIX, lipopolysaccharide-induced CXC chemokine; LECAM-1, leukocyte endothelial cell adhesion molecule 1; CCL7, chemokine (C-C motif) ligand 7; TIMP-1, tissue inhibitor of metalloproteinase 1; VEGF, vascular endothelial growth factor; IL-1α, interleukin 1α. ap<.05 compared with the C group; b,cp<.05 compared with the M group.
Immunohistochemistry analysis of lung samples
Immunohistochemistry (IHC) staining of the lung tissue revealed that TIMP-1-, IL-1α-, and CCL5-positive cells were more prevalent in the CM group, and then followed by the M group in comparison with the C group at 28 days (Fig. 2A–R). These results confirmed that hUCB-MSC-CM increased the expression of certain immunomodulating cytokines (at the protein level) in the lungs of treated animals. Three weeks after hUCB-MSC-CM transfusion, TIMP-1-, IL-1α-, and CCL5-positive cells were still observed at the transplanted lung area in the CM group. The increased levels of TIMP-1, IL-1α, and CCL5 immunoreactivity observed in the M group were statistically significant (p<.05). The increased levels of CCL5 immunoreactivity were also significant in the CM group compared with the M group (Fig. 2S).
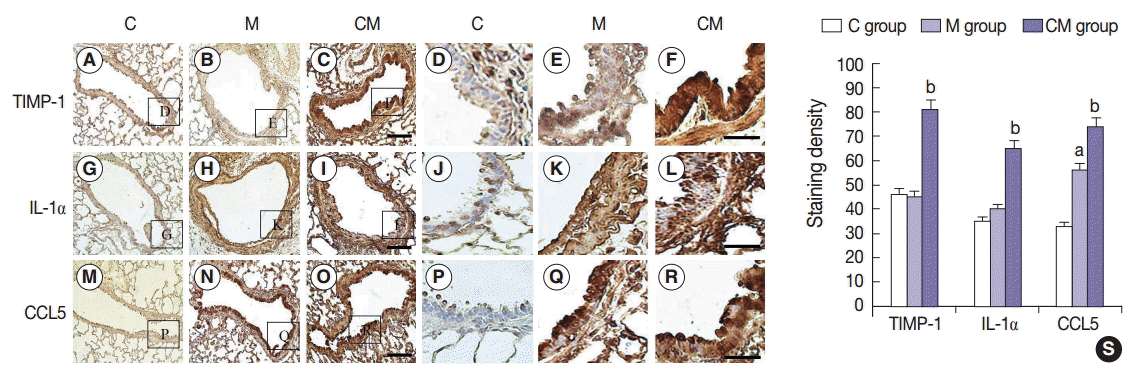
Localization of IL-1α, CCL5, and TIMP-1-immunoreactive cells in the lung tissues at 28 days. (A–R) Immunohistochemical expression reveals that the positive cells of IL-1α, CCL5, and TIMP-1 are significantly higher in the CM group than that in the C and M groups, and they are higher in the M group than that in the C group. (S) The increased levels of IL-1α, CCL5, and TIMP-1 immunoreactivity observed in the CM group are statistically significant. The levels of IL-1α, CCL5, and TIMP-1 immunoreactivity are significantly decreased in the CM group compared with the C and M groups. Panels A–C, G–I, and M–O are high power views of panels D–F, J–L, and P–R, respectively. C, control; M, monocrotaline; CM, hUCB-MSCs-CM; hUCB-MSCs-CM, conditioned medium from human umbilical-cord blood derived mesenchymal cells; TIMP-1, tissue inhibitor of metalloproteinase 1; IL-1α, interleukin 1α; CCL5, chemokine (C-C motif) ligand 5. ap<.05 compared with the C group; bp<.05 compared with the M group.
Western blot analysis
The protein expressions of CCL5 at 28 days were significantly increased in the M group compared to the C group. The protein expressions of TIMP-1, IL-1α, and CCL5 at 28 days were significantly increased in the CM group compared to the M group (Fig. 3). The protein expressions of caspase-3 and Bcl-2 were significantly increased in the M group compared to the C group at 28 days. The protein expressions of caspase-3 and Bcl-2 were significantly decreased in the CM group compared to the M group at 28 days (Fig. 4).
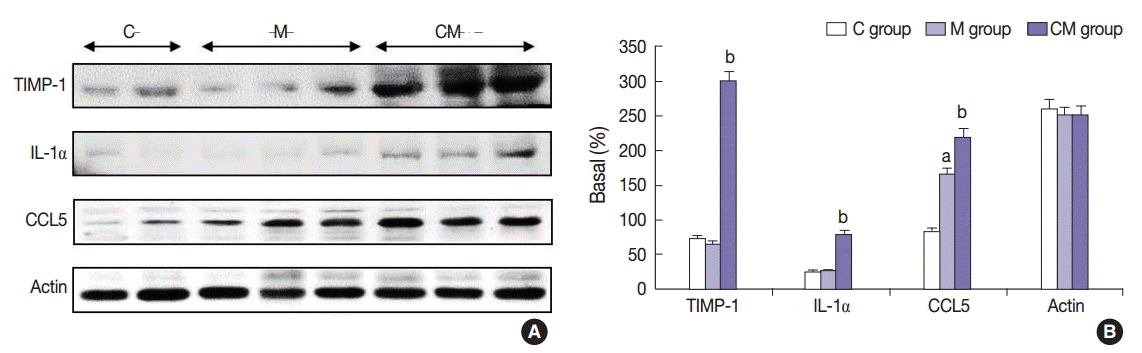
Changes of IL-1α, CCL5, and TIMP-1 protein expression levels after hUCB-MSCs-CM injection in PAH rats. (A) These are pictures of protein expression levels of IL-1α, CCL5, and TIMP-1 in the lung tissues. (B) The protein expressions levels of IL-1α, CCL5, and TIMP-1 at 28 days are significantly increased in the CM group compared to the C and M groups. The protein expressions of CCL are increased in the M group compared to the C group. C, control; M, monocrotaline; CM, hUCB-MSCs-CM; hUCB-MSCs-CM, conditioned medium from human umbilical-cord blood derived mesenchymal cells; TIMP-1, tissue inhibitor of metalloproteinase 1; IL-1α, interleukin 1α; CCL5, chemokine (C-C motif) ligand 5. ap<.05 compared with the C group; bp<.05 compared with the M group.
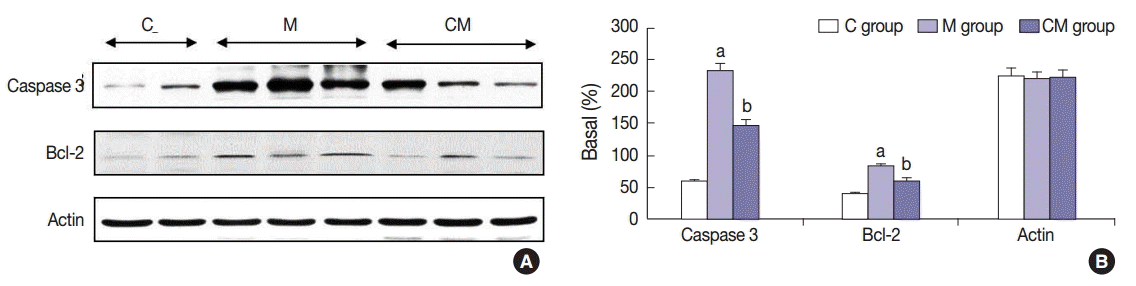
Changes of caspase-3 and Bcl-2 protein expression levels after hUCB-MSCs-CM injection in PAH rats. (A) These are pictures of protein expression levels of caspase-3 and Bcl-2 in the lung tissues. (B) The protein expressions levels of caspase-3 and Bcl-2 at 28 days are significantly increased in the M group compared to the C groups. However, the protein expressions levels of caspase-3 and Bcl-2 are decreased in the CM group compared to the M group. C, control; M, monocrotaline; CM, hUCB-MSCs-CM; hUCB-MSCs-CM, conditioned medium from human umbilical-cord blood derived mesenchymal cells. ap<.05 compared with the C group; bp<.05 compared with the M group.
TUNEL apoptosis assay
The TUNEL staining was performed to detect apoptotic DNA in the lung tissue. The assayed C group did not have any positive staining (Fig. 5A, D). However, the M group had lung tissues with a positive TUNEL staining as seen by the presence of dark brown nuclei (Fig. 5B, E). CM group also contained cells with brown nuclei, indicating apoptotic DNA (Fig. 5C, F). Apoptotic cells were significantly more prevalent in the M group than in the C group, but they were less prevalent in the CM group than in the M group (Fig. 5G). The results indicated that hUCB-MSC-CM could attenuate apoptosis in the lung tissues of treated PAH rats.
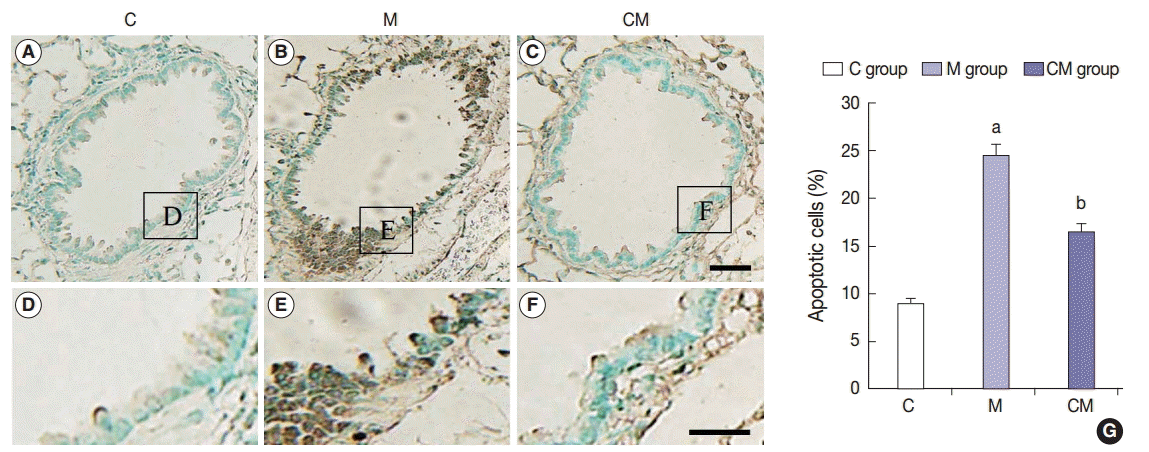
TUNEL assay of lung tissues at 28 days after hUCB-MSCs-CM transfusion. (A–F) Immunohistochemical expression reveals that the positive cells of apoptosis are significantly higher in the M group than that in the C group; however, they are lower in the CM group than that in the M group. (G) The increased levels of TUNEL immunoreactivity observed in the M group are statistically significant. The levels of TUNEL immunoreactivity are significantly decreased in the CM group compared with the M group. This result indicates that hUCB-MSCs-CM could attenuate the vascular remodeling. Panels A–C are high power views of panels D–F, respectively. C, control; M, monocrotaline; CM, hUCBMSCs-CM; hUCB-MSCs-CM, conditioned medium from human umbilical-cord blood derived mesenchymal cells. ap<.05 compared with the C group; bp<.05 compared with the M group.
DISCUSSION
In this study, we tested the effects of CM infusion on PAH affected lung tissue in a rat model. It was previously demonstrated that CM of hUCB-MSCs contain active levels of a number of disease modifying growth factors and cytokines [21,22]. CM of hUCB-MSCs contain sizable levels of angiopoietin, hepatocyte growth factor, interleukin-4, insulin-like growth factor, placental growth factor, vascular endothelial cell growth factor, angiogenin, stem cell factor, and tyrosine hydroxylase [5,23-25]. Our previous studies demonstrated the neuroprotective effects of conditioned media from hADSC and hNSC in rat models of stroke and Huntington’s disease [14,15]. Therefore, we chose to test the CM prepared from hUCB-MSCs in a PAH rat model for therapeutic signals.
MSCs are multipotent stromal cells that have self-renewal capacity, and can differentiate into a variety of cell types such as osteoblasts, chondrocytes, myocytes, and adipocytes [26,27]. MSCs have been isolated from several different sources such as embryonic tissue, bone marrow, adipose tissue, and the placenta [28]. MSCs are the source of many immune-dampening cytokines and in this regard, have demonstrated potency in a number of disease models [29,30]. In addition the secreted factors from MSCs display antiapoptotic, proliferative activity and the cells may be involved in the removal of harmful factors from their vicinity [23,31].
From our study, we detected relatively high concentrations of CCL5, TIMP-1, and IL-1α in hUCB-MSC-CM treated lung tissues compared with MCT alone (M) and control (C) groups as confirmed by a rat cytokine array panel (Fig. 1). Cytokines play important roles in a number of biological processes including innate immunity, apoptosis, angiogenesis, cell growth, and differentiation [32]. These processes play important roles in disease protection and recovery.
Lipopolysaccharide-induced CXC chemokine (also termed CXCL5) is a member of the CXC chemokine family, and is a potent neutrophil chemoattractant [33]. A TIMP-1 is a naturally occurring inhibitor of metalloproteinases [34-36], and TIMPs inhibit tumorigenesis, cellular invasion, metastasis, and angiogenesis. TIMPs may also promote tumor growth and inhibit apoptosis. These opposite roles of TIMPs in tumor regression and progression have been attributed to modulation by the tissue microenvironment [37]. Many cytokines induce endothelial cells to express adhesion molecules and lead to secretion of chemokines that attract white blood cells to a site of injury [38,39]. In our study, for the hUCB-MSC-CM treated PAH induced animals, the lung tissues showed significant increases in the number of IL-1α-positive pulmonary arterioles compared with the control group.
IL-1α (and also tumor necrosis factor α) are known to stimulate proliferation of endothelial cells and fibroblasts that increase the blood supply at the site of injury and repair damage [40]. The IL-1 family includes the structurally related proteins IL-1α, IL-1β, and IL-1 receptor antagonist that bind to the same receptor. The IL-1 family plays an important role in interstitial lung diseases. Previous research has demonstrated that IL-1α expression levels in the lung correlated with the development of pulmonary fibrosis in rodents exposed to bleomycin or radiation [41,42]. Furthermore, studies have demonstrated up-regulation of IL-1α expression in fibro-proliferative areas within the lungs of idiopathic pulmonary fibrosis patients [43]. IHC for IL-1α, CCL5, and TIMP-1 confirmed the lung cell increases for these three cytokines previously seen in the lung homogenates for the three cytokines (Fig. 2). How the characteristics of the above cytokines may either ameliorate or exacerbate the effects of PAH remain to be explored.
The numbers of TUNEL-positive cells in the lung areas were also significantly reduced by the infusion of hUCB-MSC-CM (Fig. 5). The hUCB-MSC-CM treatment was initiated 28 days after induction of PAH. Therefore, the reduction of apoptosis could be due to protective mechanisms of the hUCB-MSC-CM. These therapeutic effects could provide a clinically relevant benefit to patients. For our study, although no cells were implanted, our data demonstrated that an infusion of hUCB-MSC-CM can significantly reduce lung cell apoptosis due to PAH in our rat model. This novel therapeutic modality could be a viable treatment for PAH and bypass several technical limitations of a direct MSC cell transplantation. The present study also revealed certain changes in chemokine, cytokine, and growth factor levels after hUCB-MSC-CM transfusion in a PAH rat model. Through a complex interaction of these mediators involved in immunomodulation and inflammation, we may expect a positive effect on reducing the impact of PAH on lung cells. Exactly how these cytokines and factors interact to impact the survival of the lung tissue cells remains to be explored. As there are several treatment options available for PAH in people, an effective therapy in prolonging survival remains elusive. Our data with factors present in hUCB-MSC-CM may present an exciting opportunity for more effective therapies.
The limitations of our study included the small sample size and a short follow-up of the treated animals. Future studies with larger sample sizes and a longer duration of treatment will be required, along with standardizing the quality and amount of hUCB-MSC-CM, frequency, and the duration required for the treatment.
Notes
Conflicts of Interest
No potential conflict of interest relevant to this article was reported.
Acknowledgements
This work was supported by a Korea University grant.